designing for learner engagement / 23 designing for learner engagement in middle school science: technology, inquiry, and the
Designing for Learner Engagement / 23
Designing for Learner Engagement in Middle School Science:
Technology, Inquiry, and the Hierarchies of Engagement
Andrea J. Harmer and Ward Mitchell Cates
Lehigh University
Running Head: Designing for Learner Engagement
4086 words of body text
2 tables and 4 figures
788 words in 39 references in APA 5th edition
In today’s world of reality TV, the Internet and video games,
capturing the attention of students in science classes is becoming
increasingly difficult (Castell & Jensen, 2004). However, just
capturing students’ attention is likely not enough. The National
Center for Educational Statistics (2002) reported that, although 92.7%
of students could understand basic scientific principles, only 57.9%
could apply them, and a mere 10.9% could analyze procedures or data.
Torp and Sage (1998) argued the problem is traditional science
curricula focus on having students memorize facts rather than
constructing knowledge through active, authentic experiences. (see
also Bybee, 2003; Hurd 1991). By “authentic experiences,” the authors
appear to mean offering students the opportunity to engage in
real-world situations involving science. Others agree and advocate
scientific inquiry reform, which views science much as scientists do,
as a way of finding out why natural phenomena occur (see for example,
Dunne, Loucks-Horsley & Mundry, 2005).
The National Academy of Science (1995) suggested the most effective
way to introduce inquiry is to link it to something students already
know, and the National Research Council (1996) declared inquiry into
authentic questions generated from student experiences, to be the
central strategy for teaching science. The intent is to engage
learners in inquiry. In fact, engaging learners in science is
considered the first “essential” ingredient in a widely recognized
inquiry model, known as the “Five E’s” (Bybee, et al., 1989) and is
considered a key feature of classroom inquiry in the frequently
referenced Inquiry and the National Science Education Standards (NRC,
1996). Newmann (1986) argued engagement is difficult to define, but we
recognize it when we see it. He further argued that engaged students
care about their work and commit themselves to it because their work
seems valuable beyond the confines of the classroom.
Twenty years ago, active student engagement was reported to enhance
and increase student learning, achievement and personal development
(National Institute of Education, 1984). Ten years ago, Apple
Computer’s Classrooms of Tomorrow [ACOT] Report (1994) argued that
technology-rich classrooms produced positive changes in student
engagement, and further contended that conditions for sustaining
student engagement include using technology within the context of a
meaningful assignment, while allowing for exploration and
experimentation.
Csikszentmihalyi’s (1975) vision of “flow” creates a framework to
interpret student engagement. He defined flow as total immersion in an
activity motivated primarily by intrinsic rewards with a fine balance
between a challenging task and the possession of skills to carry out
the task. Flow is also the critical component of enjoyment. Therefore,
if flow theory is applied to engagement in the science classroom,
inquiry should be designed to be intrinsically motivating,
challenging, skill- and confidence-building, and fun. If you combine
ACOT’s contentions with flow theory, inquiry should include the use of
technology and allow for freedom of exploration within a meaningful
assignment.
Problem-based scientific inquiry, which has students investigate
science by solving a problem, may be a way to provide a meaningful
assignment and thus a way to engage learners. Shapiro (1994) contended
problem-based scientific inquiry might actively engage middle school
students in more authentic learning, promote greater knowledge
acquisition, and develop students’ problem-solving abilities. Savery
and Duffy (1996) proposed that in order to design effective
problem-based environments, the learner must “own” the problem, as
well as the process. By “owning the problem,” the authors appear to
mean that students must be able to relate to the problem enough to be
motivated to solve it.
While problem-based learning is gaining wider acceptance as a method
for teaching scientific inquiry (Barrows & Myers, 1993; Evenson &
Hmelo, 2000), the National Science Resource Center (1998) argued
middle-school learners might gain even more from these activities if
they actively engaged in designing solutions to the problems, rather
than selecting solutions from those presented within the environment.
The National Science Resource Center is not alone in advocating this
approach. Baxter MaGolda (1999) and Edelson, Gomez, and Pea (1997)
contended effective problem-based scientific inquiry must encourage
students’ self-authorship, such as designing and presenting solutions.
Solutions designed freely by students are often the result of
students’ attempts to solve “ill-structured problems,” defined by Ge
and Land (2004) as problems in which the information and actions
needed to solve the problem are not obvious.
Jennings (1995) reported middle school kids responded positively to
participating in real-life learning tasks, while Daniels (2005)
suggested middle school students’ desire to make a difference in the
world separates them from other age groups. Engaging middle school
students with real-world problems might be a way of capitalizing on
their desire to contribute. Joseph (2000) suggested we go still
further and advocated the “passion school” concept, which uses extreme
learner interest to drive learning by encouraging active engagement
with experts. Combining Csikszentmihalyi and Joseph may explain why
students who feel passion for a subject willingly invest time and
energy in it.
A recent study we conducted involving the confluence of scientific
inquiry, technology, and problem-solving with an authentic —but
ill-structured— problem provided insights into the dynamics of learner
engagement in the middle school. As a result, we derived principles
for designing for engagement we believe may apply to other subject
areas and perhaps even to other grade levels.
Materials Design
We chose as our authentic problem a public health issue, the spread of
the West Nile Virus. We produced print materials, reproduced relevant
source material, and created electron microscopy images and an
introduction to nanoscale science. This section talks a bit about how
we used technology and how we designed our materials.
We used two Web-based tools: WISE and ImagiNations. WISE (http://wise.berkeley.edu)
stands for Web-based Inquiry Science Environment and uses design
principles recommended by a number of authors for scientific
problem-solving and inquiry (see for example, Barrows & Myers, 1993;
Evenson & Hmelo, 2000; Gobert, Slotta, Pallant, Nagy, & Targum, 2002;
Linn & Hsi, 2000; Savery & Duffy, 1996). Through WISE, students
accessed information from fifteen newspaper articles about the West
Nile Virus, gathered over a two-year period (see Figure 1).
Figure 1. Screen capture from WISE Website - West Nile Virus Project
To reduce development time we adapted the WISE inquiry from a
previously prepared lesson on Malaria. In addition, we prepared and
passed out personal student journals, group folders with handouts, and
a customized CD containing full-text newspaper articles. It took
approximately one month to create all the materials.
We used ImagiNations (http://www.lehigh.edu/~inimagin) to introduce
the concept of electron microscopy and allow students access to
electron micrographs for analysis. When learners visited ImagiNations
they found an electron micrograph of a mosquito they could magnify by
clicking on it. Students could also view and download micrographs of
the West Nile Virus, a mosquito body, and human blood cells (see
Figure 2).
Figure 2. Screen capture from ImagiNations Website
Based on the suggestions from the literature, we designed the inquiry
around a community problem to which we thought students would relate.
We used phrases like, “YOU ARE A SCIENTIST TOO, WITH FRESH IDEAS!”
which we hoped would prompt students to think of themselves as
scientists. Our design encouraged ownership of team identity and
teamwork by allowing students to group themselves and choose their own
names. We encouraged the students to think creatively by allowing them
to choose their own design method and medium for both their solution
and demonstration. We provided a common foundation of resources, so
students would discuss the same material and might be able to
collaborate more easily. Being part of a scientific research community
at a nearby university, we were able to include references to
“cutting-edge” research about nanotechnology in the students’ shared
materials to foster curiosity. In this way, we intended our student
scientists to think they had special knowledge not yet available to
the general public. This also cultivated a connection with the
university scientists working on the same kinds of problems the
sixth-graders were. As a final incentive to engage students, we
discussed the brand new “aberration-corrected” microscope that had
just been assembled at the university to explore the nano world on the
atomic scale. Through the ImagiNations Website, we included images
generated from the university’s environmental scanning electron
microscope (ESEM) that related to the problem and potential student
solutions. This was to impress upon students that they had access to
the same kinds of tools scientists use to visualize microscopic
samples for solving problems.
Implementation
We then tried our materials out with a total of 55 6th grade students
in two classes in a suburban middle school in the Northeastern United
States. Their teacher volunteered to participate in what we titled the
West Nile Virus Project. Students ranged in age from 11-13, with a
mean age of 12. One class had 16 girls (57%) and 12 boys (43%), while
the other had 13 girls (48%) and 14 boys (52%). Thus, the total group
consisted of 29 girls (53%) and 26 boys (47%). Ninety-eight percent of
the students were Caucasian.
We asked students to formulate a solution for containing the deadly
West Nile Virus that had been found in their county and to design,
justify, and demonstrate their solution in a final group presentation.
The West Nile Virus Project reflected a culmination of Piagetan
principles, “hands-on” manipulation, and inquiry-based science
practices (NRC 1996; Papert, 1980, Piaget, 1967). During the first
five minutes of each class, students participated in an instructor-led
“show and tell,” which ended by passing the shown object around the
room for all to see and handle. Over a total of four weeks, covering
eight 45-minute classes, students spent a large portion of their time
using WISE and ImagiNations to learn facts about the disease, study
different solutions previously applied to the problem, and examine
microscopic samples related to the problem.
In addition to classroom problem-solving activities, we encouraged
teams to discuss their problem solving outside of class and through
online discussion in WISE during the 4-week period. We also reminded
students to write their daily thoughts in their journals.
No technology implementation is flawless, however, and our project was
no exception. For example, an automatic pretest (built into WISE)
appeared unexpectedly and confused the students. The group ID/password
log-in exercise was confusing as well and took more time than we
anticipated. In this case, each student had his or her own computer,
so it worked out better to have each student log on individually and
work offline in his or her group. Beyond the group issue, there was a
fair amount of trouble logging onto WISE once the individual IDs and
passwords were established. This happened for four reasons: 1)
bandwidth was at its most limited at the time the lesson was being
accessed; 2) students had to create two IDs and passwords (one for the
district as well); 3) all students trying to access the same location
at the same time overloaded the WISE server and hung up classroom
computers; and 4) students continually forgot their two IDs and
passwords, as well as the URLs. Despite the careful preparation of the
newspaper articles CD, students discovered it wouldn’t appear as an
icon on the school computer desktops because of district restrictions
on downloading information, which made all the CDs useless. Despite
our belief that the technology-based inquiry was manageable by one
teacher, many operational, technical, and task-related questions kept
the teacher, an aide, and the first author continuously busy.
Data Sources
In order to facilitate collecting firsthand data, the first author
served as a participant-observer (Creswell, 2003). Howe (2001) and
White (2001) suggested understanding learners’ thinking processes
requires direct exploration of their thoughts about how learning
science in school relates to themselves and to society. To explore
this, the first author interviewed student groups asking ten
questions, such as, “What makes you care about learning science?” In
addition to their daily journals and interviews, we also asked
students to complete a five-question written survey both before and
after the study. On the last day of the problem-solving activity, we
collected students’ journals and had groups present their solutions by
demonstrating them to the class. After the students’ presentations
were complete, the first author conducted a seven-question interview
with the teacher, asking her about students’ engagement with problem,
creation of student solutions, and the preparation of demonstrations.
She also observed students and teachers over the four-week period,
noting their interests, frustrations, comments, and requests.
Fortunately, we lost little data to absence: one person was absent for
the pre-treatment survey, two students missed interviews, and six
students missed making a journal entry.
Our study used three of the six strategies Merriam (1998) suggested to
enhance the internal validity of a study: triangulation, member
checks, and peer examination. To address external validity, we have
included many direct quotes and detailed narrative, as suggested by
Patton (2002).
Data Analysis
Observational data, along with student, teacher, and aide comments and
reactions, provided information about operational, technical,
managerial, class staffing and curricular issues. The students’
responses written before and after the study, along with their journal
entries, comments, and interview responses provided information about
the learning design and its effect on students’ engagement with the
technology-based inquiry. The combination of data sources created a
complex picture. It took us a long time to tease out the key
relationships.
We analyzed the data by coding the variables and putting them into
categories we constructed, as suggested by Merriam (1998). We
constructed or derived the categories from broader themes that emerged
from the variables, as suggested by Maxwell (1996). Over a three-month
period, we worked exhaustively to collapse the variables through a
rigorous data-reduction scheme, which also reduced the number of
categories. After the final reduction, seven categories remained:
1.
Personal Relevance
2.
Importance of the Problem
3.
Value of the Solution
4.
Value of Deriving the Solution
5.
Interest or Positive Attitude
6.
Student Investment of Emotions
7.
Student Investment of Time and Energy
Personal Relevance was derived from student references to how the
problem of the West Nile Virus affects them, their family members, and
where they live. Importance of the Problem was derived from student
comments that suggest they understood the seriousness of the problem,
with people getting sick or dying, while Value of the Solution
represented student references to their solutions as helpful in saving
lives and/or preventing West Nile Virus. Students’ comments and
reactions placed in Category 4 (Value of Deriving Solution) indicate
students felt they had the ability to make a difference in, or a
contribution to, stopping or slowing the spread of West Nile Virus.
This category also included student references to the importance of
the solution outside of the classroom—for example, to the community
and scientists. Examples of the types of data used in constructing the
first four categories are shown in Table 1.
Table 1. Written and Oral Student Data Classified By Data Category
(First 4 Categories)
CATEGORY
WRITTEN STUDENT DATA
ORAL STUDENT DATA
Value of Deriving Solution
--------------------------
(Making a difference, contributing to scientific knowledge, scientists
and people in community could be helped by solution)
*
“We had a chance to solve a worldwide problem.”
*
“I’m proud,”
“I feel important to be helping to solve a problem for a big
situation,”
“I’m included in doing something good for my community and
country,”
*
“to help scientists,”
“discussed ideas like presidents and governors – wicked
discussions,”
“that we are trying to help scientists,”
“makes other people know kids are thinking,”
“that we could make a difference,”
“scientists see the video – they could use our resource”
*
“Shows people what we did and what we think”
*
“maybe scientists will listen to our solutions and it will help
solve the problem” “scientists may know about it”
*
“What if solution doesn’t exist in real life?”
*
“because we are doing something good for our community”
Value of Solution Itself
(Saving people)
*
“I save a lot of people,”
“that someone
*
tries our solution and it helps”
*
“it could save lives if we can prevent it”
*
“people will die if we don’t find a solution”
Importance of Problem
(People are getting sick and dying)
*
“the president could get sick,”
”this is serious”
*
“if a kids dies that’s really sad”
*
“See, there’s like innocent people in this world and they haven’t
done anything and they could get the disease and stuff”
Personal Relevance
(Students use words indicating they are gaining information not known
previously to them or their families)
(How problem affects family members and self, and where they live)
*
“we learned a lot,”
“learning stuff I never knew,”
“that we learn from it”
*
“People who are wonderful that die from WNV and my family so that
they may be healthy and safe,” “I wouldn’t want to get infected by
it and if I’m already I would want to find a cure” “My family and
friends could get the WNV and they could be sick or even die”
*
“I didn’t know the West Nile Virus was in the Lehigh Valley.” “I
didn’t know it was at the Game Preserve.”
*
“Learning something that none of my family members knew.”
*
“Can older people get the West Nile Virus?” said in a worried
tone>
*
“Useful for hunters.”
Category 5, Interest or Positive Attitude, was derived from students’
actions and comments suggesting they were excited, motivated, focused
and eager to learn about new topics and be involved in the task.
Student Investment of Feelings and Emotions was constructed to contain
more emphatic student comments and actions about the topic and the
task. These comments suggest they were absorbed in problem solving and
were willing to invest feelings in it (for example, “I love…,” “I
hate…”). Teacher comments, such as, “they loved that” — indicating the
teacher perceived students as emotionally engaged with scientific
topic— also help to populate this category.
The final category, Student Investment of Time and Energy, was
constructed to encompass student comments indicating their desire to
take on additional work in science, anticipating they would have more
confidence in future performances. This category also reflects student
actions that indicate they were willing to invest more than just class
time in the assignment, as well as willing to give up free time to
complete the assignment, both in school and out of school (a
“sacrifice for science,” so to speak). Additionally, this category
encompasses teacher comments that report student investment of extra
time and energy to work on the task outside of school, and students’
taking interest in science home to their parents. Some student
comments and reactions placed in this category reflect the notion
suggested by Cates and Bishop (2002-03) that successful engagement in
inquiry charges learners with enthusiasm and energy for future
performances. Examples of the types of data used in constructing
categories 5 through 7 are shown in Table 2.
Table 2 Multi-source Data Classified by Category (Final 3 Categories)
Category
Observations
Written student data
Oral student data
Teacher interview data
Student Investment of Time and Energy
[“Desire for Another Run”
(Cates & Bishop, 2002-03)]
*
Students spontaneously, without prompting, described work or
progress to me as I walked around the room.
*
Several groups asked the teacher if they could come back during
activity period to work on their presentations.
*
Two groups discussed planning to meet at students’ houses on
weekend to work on solution demonstration.
*
“People will like science more now because they will have more
confidence that they can do better, if they do well on the West
Nile Virus Project.”
*
“Do you want to meet at my house to work on the video? Call me.”
*
“One group, the one with the news show, they had been up here
every single day at recess, practicing, practicing, practicing,
working on their poster every free second they got so …yeah, they
knew what they were doing.”
*
“I think they learned a lot and they were very excited about it
and a couple of parents at the conference actually talked about it
that the kids were working on it at home a lot and were motivated
by it.”
*
“Kids asked for passes for lunch recess and gave up their recesses
for a couple of recesses to come up and practice and work on
posters and stuff like that.”
*
“A lot of the kids went back and looked at the pictures; they
showed their parents the pictures, so yeah.”
Student Investment of Feelings, Emotions
*
One girl gets upset that her online comment is accidentally
submitted before she completes her thought.
*
Students are cooperative but bashful about answering interview
questions
*
“I hate all mosquitoes!”
*
“If you have a dead bird in your yard, get rid of it, those stinky
dead birds have the virus!!!!!”
*
“I love science,” “amazing,”
*
“You are stealing my solution!”
*
“We believe in our solution.”
*
“They loved that.”
*
“It was cool and they loved it.”
Interest or Positive Attitude
(focus toward topic or task)
*
Students eagerly tell one another their solutions, talking fast,
interrupting each other, using hands to describe ideas, discussion
is big and noisy, but mostly about topic, one group finds outside
sources to support their solution.
*
Students frantically put information into online comments.
*
Student quietly reprimands another during interview when one
starts goofing off while others are talking.
*
“Go to the West Nile Becomes a Fact of Life to go to the deet
site, it rocks!”
*
“We have two solutions so far.”
*
“ouuu,”
*
“interesting,”
*
“Cool,”
*
“Listen to this”
*
They were excited, motivated, eager to learn,”
*
“no pressure, they could just be creative …liked the freedom,”
*
“You could physically read their body language, even when we just
said, you know, time to go to the computer lab, they got excited”
Discussion
Although it took us quite a while to distill the seven data
categories, these tell only a small part of what we learned. According
to Chapman (2003), behavioral criteria indicate the extent to which
students make active responses to the learning task. In this study, it
appears the students’ sense of the relevance of the problem evolved on
several levels, beginning with “I wouldn’t want to get infected with
it,” and culminating with “having a chance to solve a worldwide
problem.” The range of these comments seems to indicate what we are
looking at is a hierarchy from a lower-level sense of personal
relevance to a much higher-level sense of relevance to the world
outside the classroom. Figure 3 illustrates this hierarchy,
categorizing student responses and illustrating how perception of
empowerment builds from the lowest level of personal relevance to the
highest level, value of deriving a solution.
Highest Perception
of Empowerment
Value of Deriving Solution
*
Making a difference
*
Contributing to scientific knowledge
*
Helping scientists and people in the community by solution
Value of the Solution Itself
*
Saving people
Importance of Problem
*
People are sick and dying
Personal Relevance
*
Students trying their best and learning
*
How problem affects family members, self, and where they live
Lowest Perception of Empowerment
Figure 3. Hierarchy of Empowerment in Behavioral Domain.
As the students learned facts, such as a baby in the womb could get
West Nile Virus and that the bald eagle at their local game preserve
(which many of them knew from previous field trips) died from West
Nile Virus recently, it appears they began relating to the problem
with such intensity that they gained a sense of purpose for their
inquiry. For these students, it appears it became highly relevant to
design a solution, and as a result, their solution took on more
importance. As the importance of their solution increased, it seems
the sense of their own importance increased to the point of perceived
empowerment. That is, students who reach the highest perception of
empowerment value their solution because they believe they are able to
make a difference by contributing to scientific knowledge, which could
help scientists and other people in the community and beyond. In our
study, students began to think of themselves as capable of helping
scientists and as being part of the real scientific team working on
the problem. These students felt “important,” “like presidents and
governors,” and believed that “scientists could use their resource.”
Supportive phrases designed to encourage the students throughout the
inquiry seem to have contributed to their perception of empowerment as
well. Thus, we hypothesize, learning scientific facts engages students
when those facts relate to a real purpose. A problem that is real,
local and relevant appears to provide that purpose. If students are
also encouraged to believe they are capable of contributing to the
solution of the problem (their purpose), we conclude they will engage
with the inquiry.
Additionally, student comments referring to the transfer of their new
knowledge from the classroom to the university to the community seem
to indicate the design features, such as students’ indirect connection
with scientists at the university (through the first author visits)
and the cutting-edge nature of the research are important factors
contributing to the students’ sense of empowerment. There was even
some small suggestion that access to scientists’ tools contributed to
the students’ sense of empowerment. As one student responded, “If I
hadn’t seen the picture, I wouldn’t have gotten the idea,” which seems
to show that visualization of the micrograph may have engaged him by
stimulating his thought process during the inquiry.
Affective criteria gauged the level of students’ investment in and
emotional reactions to the learning task, as well as their interest or
positive attitude (Chapman, 2003). During the inquiry, it appears what
began with a positive attitude toward the task (“Go to the Deet site;
it rocks!”) evolved into students’ more intense investment in the task
(“Do you want to meet at my house [this weekend] to work on the
video?” [their solution demonstration]). Additionally, the teacher
comment that students were using “every free second” on their
demonstration preparation indicates that students were investing much
free time in the inquiry. We interpret this to mean that students who
are willing to invest a noticeably large amount of time to the task
are exhibiting a substantially high level of interest in the inquiry.
The range of the student comments, along with their actions, seem to
indicate that what we are looking at is a hierarchy from a lower-level
sense of interest and investment to a much higher-level of interest,
which includes investment of emotion, time, and energy outside of the
classroom. It appears the students who reach the highest level of
investment grow (in Joseph’s terminology) passionate about completing
their solutions. This passion again appears to be stimulated by
designing around an authentic and relevant problem, and the students’
desire to help. We were pleasantly surprised and impressed by the
sixth-graders’ passion and determination to help others. Figure 4
illustrates this second hierarchy, categorizing students’ interest,
investment in and emotional reactions to the learning task, and
suggests how a sense of passion builds from the lowest level of
investment, a positive attitude, to the highest level, indicated by
increased confidence in scientific problem-solving and the energy to
begin and pursue additional inquiries (or, in Cates and Bishop terms,
“a desire for another run.”).
Highest Level of Investment
(Passion)
Student Investment
*
Investment of time & energy
*
Investment outside of school
*
Desire for another inquiry
Student Investment
*
Investment of feelings, emotions
Interest or Positive Attitude
*
Focus toward topic or task
Lowest Level of Investment
Figure 4. Hierarchy of Passion in Affective Domain
A key factor that contributed to the students’ high level of
commitment may have been freedom to make choices without penalty.
Students could design their solutions in any way that used at least
three facts and they were free to choose the presentation medium for
their demonstration, such as a poster or video. In addition, the
teacher chose this as an ungraded assignment, which may have also
enhanced the students’ perception of freedom for creativity without
consequence.
Student collaboration was also an important component in our study. In
fact, according to student response, collaboration was the most
helpful aspect of problem solving. We observed evidence of this:
students discussing tasks excitedly, sharing resources, thinking
aloud, “one-upping” each other with new solutions, and even finishing
each other’s sentences. A design feature that appears to have helped
contribute to this finding was the use of shared student resources
during problem-solving that allowed students to analyze the same set
of facts from their different perspectives. In addition, the
computer-based nature of the inquiry contributed to the students’
engagement by allowing students to share and interact easily within an
environment familiar to them.
As noted earlier, many educators and researchers agree problem
authenticity and relevancy, as well as having students design
solutions, are important factors for engaging students in scientific
inquiry. Findings from this study seem to confirm this. However,
additional learning design elements, which encourage empowerment,
passion and freedom of expression should not be overlooked, and may
well be as important as inquiry features already accepted as
effective. In addition, university relationships, which can foster
involvement with cutting-edge research and instrumentation may prove
effective “attention getters” for student engagement.
Application to Other Subject Areas
To what extent, however, might what we have learned in this study be
applied to other subject areas? We have generalized what we feel may
be principles that would apply across content areas –and perhaps
across grade levels— although only further study can confirm this
fact. Our ten principles follow:
1.
Choose a problem that is authentic and allows for many possible
solutions.
2.
Look for problems that have broader societal impact and whose
solution would have immediate value.
3.
As much as possible, emphasize how the problem may affect
students, their friends and families.
4.
Look for ways to utilize cutting-edge problems and to connect with
those who are working on solutions to such problems.
5.
Provide students with many options and choices as a way to
encourage their commitment.
6.
Use technology to enrich data sources, resources, and
opportunities to connect with the world outside the classroom.
7.
Employ collaboration where possible to encourage students to
engage both socially and intellectually.
8.
Use language in materials and in class that emphasizes students’
ability to accomplish something important if they try (the
vocabulary of empowerment).
9.
Enable students to work on their own outside of school on
solutions.
10.
Emphasize that student solutions will be shared with those outside
the school who are working on solutions to the problem under
study.
While our own research has focused on scientific inquiry, we can
easily envision social problems well suited to our method. We welcome
collaborative research and look forward to learning how well our
principles apply —or don’t— to other subject areas or grade levels.
References
Barrows, H.S., & Myers, A.C. (1993). Problem-based learning in
secondary schools. Unpublished manuscript. Springfield, IL:
Problem-Based Learning Institute, Lanphier High School and Southern
Illinois University Medical School.
Baxter Magolda, M.B. (1999). Creating contexts for learning and
self-authorship: Constructive-developmental pedagogy. Nashville, TN:
Vanderbilt University.
Bybee, R.W., et al. (1989). Science and technology education for the
elementary years: Frameworks for curriculum and instruction.
Washington, DC: National Center for Improving Instruction.
Bybee, R. W. (2003). Science curriculum reform in the United States.
Washington, DC: National Academy of Sciences. Retrieved May 5, 2003,
from http://www.nas.edu/rise/backg3a.htm
Castell, S., & Jenson, J. (2004). Paying attention to attention: New
economies for learning. Educational Theory, 54, 381-397.
Cates, W.M., & Bishop, M.J. (2002-2003). Learner as bobsled operator:
The physics of learner engagement. Journal of Educational Technology
Systems, 31, 291-305.
Chapman, E. (2003). Alternative approaches to assessing student
engagement rates. Practical Assessment, Research, & Evaluation.
Retrieved November 1, 2004 from
http://pareonline.net/getvn.asp?v=8&n=13.
Csikszentmihalyi, M. (1975). Beyond boredom and anxiety: Experiencing
flow in work and play. San Francisco: Jossey-Bass.
Creswell, J. W. (2003). Research design: Qualitative, quantitative and
mixed methods approaches. Thousand Oaks, CA: Sage.
Daniels, E. (2005). On the minds of middle schoolers. Educational
Leadership, 62(7), 52-54.
Dunne, K., Loucks-Horsley, S., & Mundry, S. (1998). Inquiry is a
“hands-on” and “minds-on” experience for both students and teachers:
An interactive Q&A with experts on professional development for
science teachers (Science Education Reform Dialogue). Harvard
Education Letter. Retrieved January 5, 2005 from
http://www.edletter.org/past/issues/1998-so/rf-dialogue.shtml
Edelson, D.C., Pea, R.D., & Gomez, L. (1997). Constructivism in the
collaboratory. In B.G. Wilson (Ed.), Constructivist learning
environments: Case studies in instructional design (pp. 151-164).
Englewood Cliffs, NJ: Educational Technology.
Evensen, D.H., & Hmelo, C.E. (2000). Problem-based learning: a
research perspective on learning interactions. Mahwah, NJ: Lawrence
Erlbaum.
Ge, X., & Land, S.M. (2004). A conceptual framework for scaffolding
ill-structured problem-solving processes using question prompts and
peer interactions. Educational Technology Research & Development, 52(2),
5-22.
Gobert, J., Slotta, J., Pallant, A., Nagy, S., & Targum, E. (2002). A
WISE inquiry project for students’ east-west coast collaboration.
American Educational Research Association Inquiry Paper. Retrieved
March 26, 2003, from
http://mtv.concord.org/publications/online_learning.pdf
Howe, K.R. (2001). Qualitative educational research: The philosophical
issues. In Handbook of research on teaching (4th ed., pp. 201-208).
Washington, DC: American Educational Research Association.
Hurd, P.D. (1991). Why we must transform science education.
Educational Leadership, 49, 33-35.
ImagiNations (2001). What is electron microscopy? Retrieved February
28, 2005 from http://www.lehigh.edu/%7Einimagin/whatisem.html
Jennings, J.F. (1995). School reform based on what is taught and
learned. Retrieved September 10, 2004 from
http://www.ctredpol.org/pubs/reform.html
Joseph, D.M. (2002). Passion as a driver for learning: A framework for
the design of interest-centered curricula. Seattle, WA: John G.
Nicholls Trust. Retrieved February 28, 2005 from
http://tigger.uic.edu/~thork/Trust/joseph.htm
Linn, M.C., & Hsi, S. (2000). Computers, teachers, peers: Science
learning partners. Hillsdale, NJ: Erlbaum.
Maxwell, J.A. (1996). Qualitative research design: An interactive
approach. Thousand Oaks, CA: Sage.
Merriam, S. B. (1998). Qualitative research and case study
applications in education. San Francisco, CA: Jossey-Bass.
National Academy of Sciences (1995). National science education
standards (draft). Washington, DC: National Academy Press.
National Center Educational Statistics (2002). Percent of student at
or above selected science proficiency levels by sex, race/ethnicity,
control of school, and age: 1977 to 1999. Retrieved March 25, 2003,
from http://www.nces.ed.gov/pubs2002/digest2001/tables/dt131.asp.
National Institute of Education (NIE) Study Group on the Conditions of
Excellence in Higher Education (1984). Involvement in learning:
Realizing the potential of higher education. Washington, D.C.: NIE.
National Resource Council (1996). National science education standards.
Washington: DC: National Academy Press.
National Science Resource Center (1998). Resources for teaching middle
school science. Washington, DC: National Academy. Retrieved March 26,
2003, from http://books.nap.edu/books/0309057817/html/R3.html#pagetop
Newmann, F. (1986). Priorities for the future: Toward a common agenda.
Social Education, 50, 240-250.
Patton, M. Q. (2002). Qualitative research & evaluation methods.
Thousand Oaks, CA: Sage.
Piaget, J., & Inhelder, B. (1967). The coordination of perspectives.
In the child’s concept of space (pp. 209-246). New York: Norton.
Papert, S. (1980). Mindstorms. New York, NY: Basic Books.
Sandholtz, J. H., Ringstaff, C., & Dwyer, D.C. (1994). Student
engagement revisited: Views from technology-rich classrooms. Apple
Classrooms of Tomorrow Report #21. Cupertino, CA: Apple Computer, Inc.
Savery, J.R., & Duffy, T.M. (1996). Problem-based learning: An
instructional model and its constructivist framework. Educational
Technology, 35(5), 31-38.
Shapiro, B.L. (1994). What children bring to light: A constructivist
perspective on children’s learning in science. New York: Teachers
College.
Torp, L., & Sage, S. (1998). Problems as possibilities. Alexandria,
VA: Association for Supervision and Curriculum Development.
Web-based Inquiry Science Environment -WISE (2000). Retrieved June 7,
2005, from http://wise.berkeley.edu/
White, R. (2001). The revolution in research on science teaching. In
handbook of research on teaching (4th ed., pp. 457-471). Washington,
DC: American Educational Research Association.
Copyright 2005 ◦ AJ Harmer and Ward Mitchell Cates
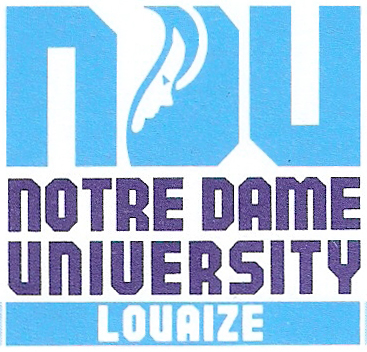
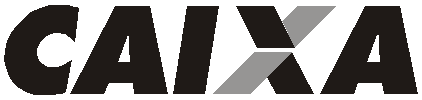
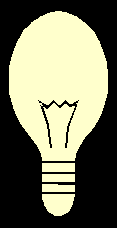


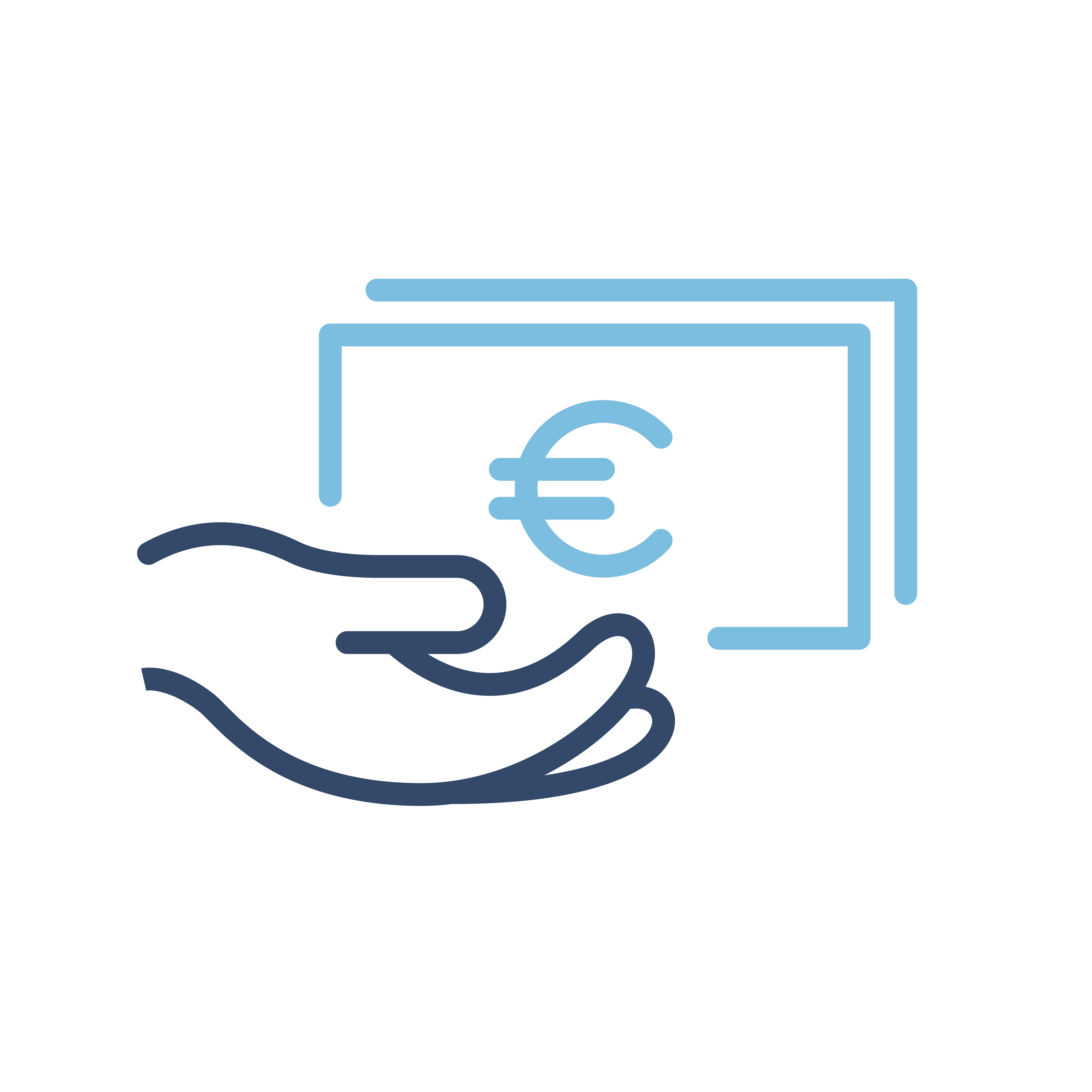
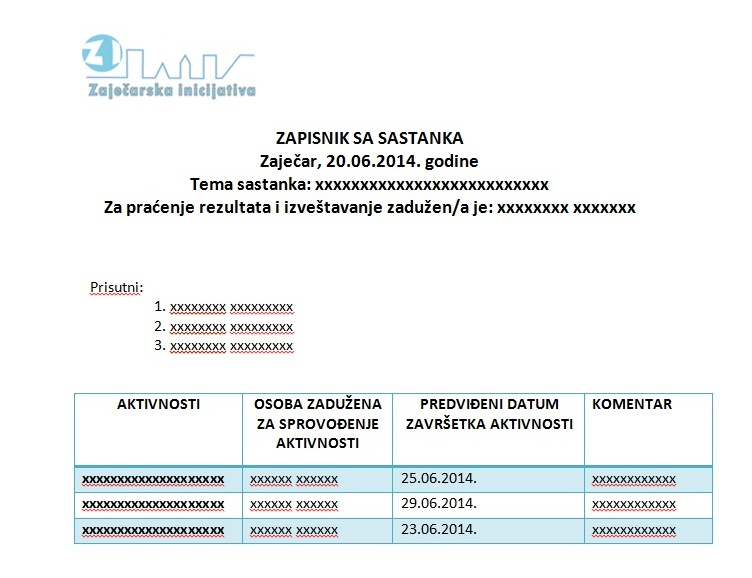
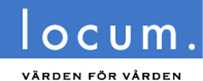

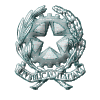
